Infant Brain Differences Linked to Autism
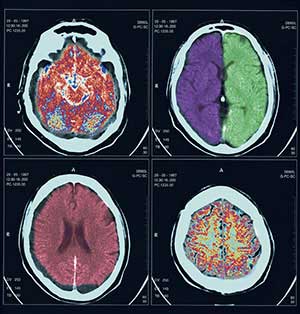
Rather than appearing suddenly in young children, autism develops over time, according to a recent study by the Infant Brain Imaging Network, a nationally funded research network with four primary sites, including the Center for Autism Research at The Children’s Hospital of Philadelphia. The investigators found significant differences in brain development starting at age 6 months in high-risk infants who later develop autism. The finding is important because it brings scientists one step closer to developing a biomarker to assist with very early diagnoses for autism spectrum disorders.
The investigators studied 92 infants considered to be at high risk for autism spectrum disorder (ASD) because they all have older siblings with autism. Each infant underwent diffusion tensor imaging — a type of magnetic resonance imaging — at 6 months and behavioral assessments at 24 months. Most of the children also had additional brain imaging scans at either or both 12 and 24 months.
At 24 months, 28 infants (30 percent) met criteria for ASDs while 64 infants (70 percent) did not. The two groups differed in white matter fiber tract development — pathways that connect brain regions — as measured by fractional anisotropy (FA). FA measures white matter organization and development, based on the movement of water molecules through brain tissue.
This study examined 15 separate fiber tracts, and found significant differences in FA growth trajectories in 12 of the 15 tracts between infants who did develop autism versus infants who did not. Infants who later developed autism had elevated FA at six months but then experienced slower development over time. By 24 months of age, infants with autism had lower FA values than infants without autism.
Intensive early intervention has been shown to improve outcomes in children with developmental delays and autism. The research therefore raises the possibility that healthcare givers may be able to intervene even before a child is 6 months old, to blunt or prevent the development of some autism symptoms.
New Stem Cells Provide Safe Study Source
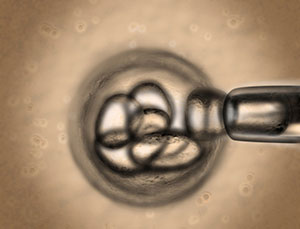
CHOP researchers have generated a new type of human stem cell that can develop into numerous types of specialized cells, including functioning pancreatic beta cells that produce insulin. Called endodermal progenitor (EP) cells, the new cells show two important advantages over embryonic stem cells and induced pluripotent stem cells: they do not form tumors when transplanted into animals, and they can form functional pancreatic beta cells in the laboratory.
“Our cell line offers a powerful new tool for modeling how many human diseases develop,” said study leader Paul J. Gadue, PhD, a stem cell biologist at Children’s Hospital. In addition to producing beta cells, the researchers also directed EP cells to develop into liver cells and intestinal cells — both of which normally develop from the endoderm tissue layer early in human development.
The researchers manipulated two types of human stem cells — embryonic stem cells (ESCs) and induced pluripotent stem cells (iPSCs) — to become EP cells. Because both stem cell populations proliferate in great numbers and potentially generate all types of tissue, they offer enormous promise for scientists to precisely control cell development, both for the study of basic biology and for future cell-based treatments.
In the current study, the researchers used signaling molecules called cytokines to steer ESCs and iPSCs into becoming EP cells, committed to developing into endoderm, one of the three tissue layers found in early human development. The EP cells have nearly unlimited potential for growth in the laboratory. Both in cell cultures and when transplanted into animals, the team showed that EP cells can differentiate into multiple cell types, representing those found in the liver, pancreas, and intestine. Importantly, undifferentiated EP cells did not form teratomas in the team's transplantation studies.
However, Dr. Gadue stressed that these early results are only the first steps in researching EP cells. Further work may focus on taking cells from individual patients with genetic forms of diabetes or liver disease to derive EP cell lines. And although applying this science to cell therapy is years away from practical clinical use, EP cells may offer a powerful starting point for developing tissue replacement treatments.
Gene Therapy for Blindness Shows Success
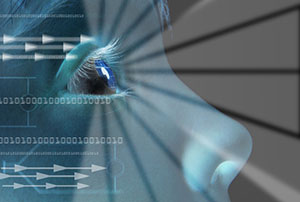
Gene therapy for congenital blindness has taken another step forward, as researchers recently improved vision in three adult patients previously treated in one eye. After receiving the same treatment in their other eye, the patients’ ability to see in dim light increased, and two were able to navigate obstacles in low-light situations.
Neither the first treatment nor the readministered treatment triggered an immune reaction that cancelled the benefits of the inserted genes, as has occurred in human trials of gene therapy for other diseases. The current research targeted Leber congenital amaurosis (LCA), a retinal disease that progresses to total blindness by adulthood.
“Patients have told us how their lives have changed since receiving gene therapy,” says study co-leader Jean Bennett, MD, PhD, F.M. Kirby professor of Ophthalmology at the University of Pennsylvania. “They are able to walk around at night, go shopping for groceries and recognize people's faces — all things they couldn't do before. At the same time, we were able to objectively measure improvements in light sensitivity, side vision and other visual functions.”
Other objective results came from brain signals seen in neuroimaging. When a dimly flickering checkerboard pattern flashed in front of a patient's recently treated eye, an area in the brain responsible for vision lit up during functional magnetic resonance imaging (fMRI). “This finding is telling us that the brain is responding to the eye’s sensitivity to dim light,” said radiology researcher and study co-leader Manzar Ashtari, PhD, of Children’s Hospital.
Furthermore, Dr. Bennett adds, the research holds promise for using a similar gene therapy approach for other retinal diseases. Dr. Ashtari said that fMRI could play a future role in helping to predict patients more likely to benefit from gene therapy for retinal disease.
Green Tea Could Treat Metabolic Disease
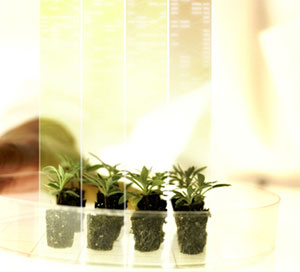
Green tea has been the subject of numerous research studies in the last decade in an effort to determine whether the tea can ward off diseases like cancer or heart disease. A CHOP endocrinologist is now exploring whether green tea may be used to treat a rare childhood disease.
Charles Stanley, MD, is an internationally prominent expert on forms of congenital hyperinsulinism (HI), rare inherited disorders in which children produce abnormal levels of insulin. He also discovered a syndrome in which HI is combined with hyperammonemia (HA), an abnormal level of blood ammonia.
In HI/HA, the mutation that he identified prevents an enzyme called GDH from functioning normally, leading to excessive levels of insulin, as well as childhood epilepsy, seizures and learning disabilities. Current drug treatment controls insulin levels, but not the neurological problems. The drug also has side effects, such as excessive hair growth.
Based on a decade-long scientific collaboration, Dr. Stanley co-authored a recent study showing that a compound found in green tea controls this enzyme defect in animals, raising the possibility of a new treatment for HI/HA in children. The study, which appeared in the Journal of Biological Chemistry, showed that the compound controlled blood sugar levels in the animals.
“Our research doesn’t mean that people should automatically expect green tea to treat the rare disease HI/HA,” says Dr. Stanley. “However, with further research, we may be able to modify this green tea compound to derive a drug that can be tested in children with HI/HA. It’s possible that such a derivative may control blood sugar levels in people, as successfully as in mice, with fewer side effects than existing drugs. An even more exciting prospect is that this may lead to a drug that is more effective than existing drugs in treating neurological problems in the disease.”
Newborn Period May Be Crucial to Preventing Diabetes
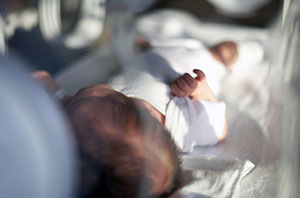
Children’s Hospital researchers who tested newborn animals with an existing human drug used in adults with diabetes report that this drug, when given very early in life, prevents diabetes from developing in adult animals. If this finding can be repeated in humans, it may become a way to prevent at-risk infants from developing type 2 diabetes.
“We uncovered a novel mechanism to prevent the later development of diabetes in this animal study,” says senior author Rebecca A. Simmons, MD, a Children’s Hospital neonatologist. "This may indicate that there is an important developmental window, a period of time in which we can intervene to permanently protect the body's insulin-producing cells."
Dr. Simmons and lead author Sara E. Pinney, MD, a pediatric endocrinologist at Children’s Hospital, published the study in the October issue of Diabetologia. The research may be relevant to children with intrauterine growth retardation (IUGR), a common complication during the mother's pregnancy. Simmons’s previous research showed that IUGR, which is associated with decreased availability of nutrients and hormones to the developing fetus, permanently alters gene expression and impairs the function of insulin-producing cells in the pancreas. These defects have been shown to cause type 2 diabetes to develop in adulthood.
The study team used exendin-4 (Ex-4), a drug recently approved for use in adults with type 2 diabetes — a condition in which a patient produces insufficient insulin, or is unable to process insulin normally. In their study, Drs. Simmons and Pinney used rats with an induced form of IUGR. They found that after being given to newborn rats, Ex-4 had epigenetic effects — modifying gene function without changing the underlying DNA sequences. Ex-4 increased the expression of a gene called Pdx1 that is necessary for beta cells to function properly. Beta cells produce insulin in the pancreas of mammals, including humans.
“In our study, giving exendin-4 during the newborn period had permanent beneficial effects on beta cells,” says Dr. Pinney. “This could be important for people, in whom abnormal changes in infancy may irreversibly alter beta cells and lead to adult-onset diabetes.”
Study Improves Understanding of Mitochondria
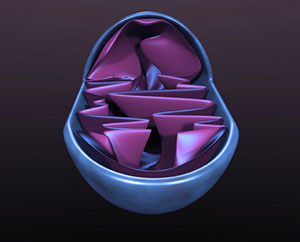
A new study by Children’s Hospital researchers answers a long-standing question about how mitochondrial DNA figures in the production of cellular energy. The study, which was led by Neal Sondheimer, MD, PhD, improves our understanding of how mitochondria work, and could eventually lead to new ways of treating mitochondrial diseases.
The mitochondrion is a key supplier of the energy needed for the multiple functions of our cells. These organelles play a pivotal role in human health, because when the mitochondrial power plants of our cells become damaged the energy output for the body's cells and tissues progressively declines. Mitochondria contain their own DNA, called mitochondrial DNA (mtDNA). The purpose of Dr. Sondheimer’s study was to determine how mtDNA is used to create proteins used in the generation of cellular energy.
In order to make new proteins, mtDNA must be converted, or transcribed, into mitochondrial RNA (mRNA). But exactly how mtDNA does this has been a source of some controversy. While some investigators suggested the existence of a second heavy strand promoter — a specialized segment of DNA required for RNA synthesis — some researchers doubted the second promoter's existence because they couldn't get it to work in the lab.
In addition to determining how to make the promoter, known as HSP2, work, Dr. Sondheimer and his team identified several of its key features. The most important and “unexpected” feature of HSP2 is that a protein that normally activates mitochondrial promoters, transcription factor A (TFAM), acts to block transcription at HSP2. The study team's finding “suggests that the cell may manipulate TFAM levels to change the genes that are expressed by the mitochondrion,” Dr. Sondheimer said.
Because determining the inner workings of the mitochondria could provide a major new approach to understanding and developing therapies for myriad rare and common diseases, the study’s findings could have significant repercussions.
Virus Mimics Cell Signals to Drive Infection
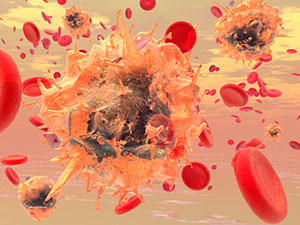
New research reveals how an invading virus hijacks a cell's workings by imitating a signaling marker to defeat the body's defenses. By manipulating cell signals, the virus destroys a defensive protein designed to inhibit it. This finding may represent a broader targeting strategy used by other viruses, and could lay the groundwork for developing more effective treatments for infectious diseases.
“Learning details of how cells respond to viruses helps us to understand key cellular machinery better,” said CHOP investigator and study leader Matthew D. Weitzman, PhD.
Biologists have long known that viruses hijack cellular processes to replicate themselves, and that host cells have evolved defense systems to resist viral invasion. To replicate, viruses must deliver their own DNA into a cell’s nucleus, so a viral infection entails a conflict between two genomes — the DNA of the host cell versus the foreign DNA of the virus. This study focused on herpes simplex virus type-1 (HSV-1), a common human virus that results in recurrent infections alternating with inactive periods. Like other viruses, HSV-1 is known to manipulate cellular processes in order to infect cells, but the specific mechanisms by which it acts on the DNA repair pathway were previously unknown.
Dr. Weitzman’s study team was studying a viral protein called ICP0 that overcomes host defenses by targeting cellular proteins for destruction. They found that ICP0 exploits phosphorylation, a chemical mark that is often used in cells to promote interactions between proteins, especially as part of the cellular signaling response to DNA damage. In HSV-1 infection, the phosphorylation signal on ICP0 attracts a cellular DNA damage response protein, RNF8, which binds to the false signaling marker and is then degraded. Because RNF8 normally inhibits viral replication, its destruction leaves the cell vulnerable to HSV-1 infection, as the virus takes over the cell's machinery.
The investigators also found that ICP0 exploits the same phosphorylation signal to bind to other cellular proteins in addition to RNF8, a hint that it may play a broader role in defeating antiviral defenses and manipulating cellular machinery. Dr. Weitzman plans to continue to investigate HSV-1 infection in neurons and in animal models, eventually extending his research into other viruses, which may act on different pathways than HSV-1 does.
Asthma Measures Do Not Reduce Hospital Visits
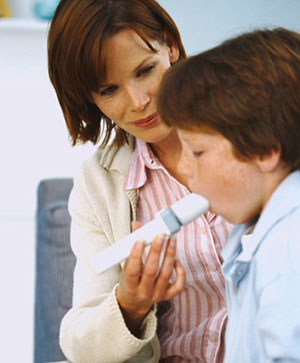
Asthma remains the major cause of preventable hospitalizations for children in the U.S. A recent study in the Journal of the American Medical Association is the first to look at the association of asthma care quality measures during hospitalization and subsequent asthma-related readmissions or emergency care.
The three Children’s Asthma Care (CAC) core measures are monitored by the Joint Commission and are meant to evaluate whether patients aged 2 to 17 years with an asthma exacerbation received relievers and systemic corticosteroids during their stay, and whether they were discharged with a complete plan of care for at-home management of asthma. Evan Fieldston, MD, MBA, MSHP, a pediatrician and health services researcher at PolicyLab, was one of several investigators to collaborate on this multi-center study.
The study included data from 30 freestanding children’s hospitals in the United States. Researchers found that, while compliance for the three CAC core measures increased over the 2-year study period, improved compliance was not associated with declines in returns to the emergency department or hospital readmission in the 7, 30, and 90 days that followed an initial hospitalization for asthma.
While home management plans of care may be an important component of treatment of asthma, the authors conclude that they may not be appropriate to classify as “accountability measures” for hospitals given that hospital-based physicians cannot monitor what happens to a family once they leave the hospital and that so much of what happens to a patient with asthma is influenced by exposures beyond the walls of the hospital.
Although post-discharge education and follow up is an important aspect of care to measure, the lack of an association between this specific measure and readmission highlights an opportunity for further investigation and refinement. The research team recommended that the asthma home management plan of care be re-evaluated as an accountability measure used by the Joint Commission, with the understanding that the key is to ensure that evidence-based practices are part of the plan families follow at home and that the plan is communicated effectively to enhance adherence.
Genome Editing Corrects Hemophilia in Animals
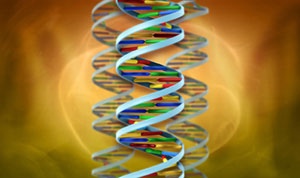
Using an innovative gene therapy technique called genome editing that hones in on the precise location of mutated DNA, scientists have treated the blood clotting disorder hemophilia in mice. This is the first time that genome editing, which precisely targets and repairs a genetic defect, has been done in a living animal and achieved clinically meaningful results.
As such, it represents an important step forward in the decades-long scientific progression of gene therapy — developing treatments by correcting a disease-causing DNA sequence.
In this new study, published in the journal Nature, researchers used two versions of a genetically engineered virus called adeno-associated virus, or AAV — one carrying enzymes that cut DNA in an exact spot and one carrying a replacement gene to be copied into the DNA sequence. All of this occurred in the liver cells of living mice.
“Our research raises the possibility that genome editing can correct a genetic defect at a clinically meaningful level after in vivo delivery of the zinc finger nucleases,” said the study leader, Katherine A. High, MD, a hematologist and gene therapy expert at Children’s Hospital. Dr. High, a Howard Hughes Medical Institute Investigator, directs the Center for Cellular and Molecular Therapeutics, and has investigated gene therapy for hemophilia for more than a decade.
Chromatin Loop Manipulation May Offer Treatments
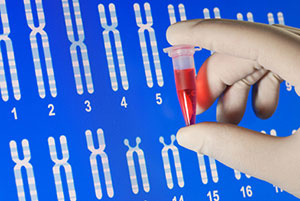
By exploring how proteins interact with DNA sequences to regulate gene activity, CHOP researchers have shed light on biological processes that could be manipulated to provide new disease treatments. In a cell’s nucleus, elements in DNA called promoters and enhancers communicate with each other while carrying out gene activity, and as these elements come into physical contact, the intervening DNA sequences bend into loops made of chromatin fiber — the substance of chromosomes.
“Many researchers … have shown that chromatin looping is widespread during gene expression,” said study leader Gerd A. Blobel, MD, PhD, of the Division of Hematology. “However, many details remain uncertain — even whether chromatin loops are a cause or effect of gene transcription.”
The researchers focused on gene transcription — the process by which DNA is converted into RNA to make new proteins — studying a portion of DNA called the beta-globin locus that expresses part of the hemoglobin molecule. While they understood the mechanics of how chromatin loops form, the investigators did not know all the proteins that were necessary to generate chromatin loops, nor how those proteins interacted with other proteins during gene transcription.
The team sought to identify a looping factor, a protein that triggers chromatin looping. “We had a strong candidate for a looping factor — a molecule called Ldb1,” noted first author Wulan Deng. In the current study, Dr. Blobel’s team used a genetically-engineered DNA binding protein called a zinc finger (ZF) protein, which was designed to latch onto a chosen gene location. The researchers attached Lbd1 to a ZF, ultimately causing a chromatin loop to form between the enhancer and promoter, and allowing high-level gene transcription to occur.
In addition to showing that Lbd1 plays a key role in gene expression, the “results suggest that chromatin looping is a cause, not an effect, of gene transcription. We will further study whether and how we can use forced chromatin looping to manipulate gene expression for scientific or therapeutic purposes,” Dr. Blobel said.
Though the findings have no immediate clinical impact, the work could lead to a number of future applications, such as treating sickle cell anemia. And more broadly, forced chromatin looping might enable researchers to turn off the expression of specific genes known to drive particular diseases, Dr. Blobel added.
Genetic Mutations Found to Impair Childhood Growth
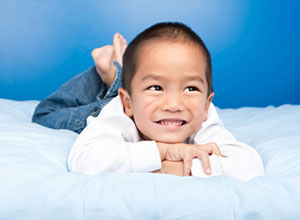
CHOP investigators studying rare genetic disorders have gained insights into biological structures that regulate chromosomes when cells divide. The researchers found mutations that disrupt the cohesion complex, a group of proteins forming a bracelet encircling chromosome pairs, cause a recently recognized class of diseases called cohesinopathies.
The cohesin complex is associated with Cornelia deLange syndrome (CdLS), a genetic disease affecting an estimated 1 in 10,000 children. The disease has a range of severity, but classically includes mental retardation, impaired growth, heart defects, and distinctive facial features. Children’s Hospital researchers were the first to discover gene mutations that cause CdLS, and the current study identified another gene, RAD21, that when mutated causes mild cognitive and physical impairments.
After performing an analysis of children with typical CdLS and overlapping features of the disease, the researchers found that none of the children had mutations in the three genes already known to cause CdLS. They then identified a boy with a deletion in a section of chromosome 8 that contains the RAD21 gene, which was known to express a cohesin protein but not previously associated with CdLS. As an infant, the boy had been diagnosed with facial features resembling CdLS, and subsequently experienced growth retardation, but had normal cognitive development.
The researchers then focused on additional children with RAD21 deletions or mutations within the gene, and found a similar pattern — physical features, such as short stature and distinctive facial features, but with only minor cognitive delays. “These findings suggest that children who are very mildly affected may go undiagnosed,” noted study leader Matthew A. Deardorff, MD, PhD, a specialist in pediatric genetics at Children’s Hospital.
While the team’s studies investigating the molecular mechanisms involved in cohesin disorders were illuminating, the research does not currently explain the full sequence of molecular events, and further studies will investigate knowledge gaps in the process. But because the cost of whole-genome sequencing is rapidly dropping, researchers may eventually discover additional genes involved in cohesinopathies, offering further clues to how these diseases function in human development.
$2.5 Million Gift Supports Groundbreaking Autism Research
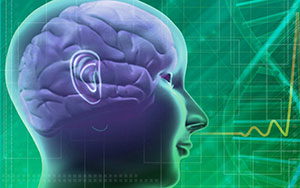
Christina Weiss Lurie and Jeffrey Lurie, Philadelphia Eagles owners and co-founders of the Lurie Family Foundation, made a $2.5 million gift to CHOP’s Center for Autism Research (CAR) in 2011. The gift supports genetic and brain imaging research to enable earlier diagnoses and more effective treatments for autism spectrum disorders (ASDs) that will help families of children with ASDs.
The gift supports two distinct lines of research. The majority will fund CAR investigators examining genetic samples from 1,000 individuals with an ASD, to look for new genetic variants that might be related to an ASD. As part of this one-of-a-kind study, families will have the option of receiving individualized genetic test results and the opportunity to discuss the results with a genetic counselor.
This information may help families as they consider future treatments, interventions, and family planning decisions.
The remainder of the gift will support research using MEG technology (magnetoencephalography) at the Lurie Family Foundation’s MEG Imaging Center at CHOP. This study will follow school-aged children over time as they develop, and will measure the brain’s activity in real time in order to better understand brain development and differences in the way the brain processes signals in children with ASD.
“We are especially excited about the possibility of using genetic information to better understand which treatments are most effective for each child and to develop better treatments in the future,” says CAR director Robert Schultz, PhD. “This requires developing a better foundation of knowledge about genes, brain functions, specific symptoms, and treatment outcomes. We hope that this kind of gift will enable us not only to determine who is at greatest risk for an ASD, but also to be able to begin to develop personalized interventions based on more precise understanding of the different causes.”
Grant Awarded to Examine Personalized Genetic Data
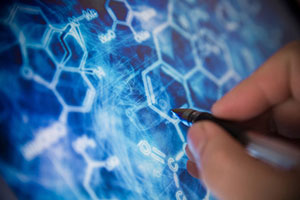
After a decade of work and at an expense of nearly $3 billion, the sequencing of the entire human genome was completed in 2003. Technological advances since then mean that an individual's genome can now be sequenced in only a few months, for about $4,000.
Knowing just what to do with this knowledge has not kept pace with the gusher of genetic data. People can now have their own genome analyzed, which can offer clues to their current and future risks of genetic diseases. But what will individuals do with this flood of information? Is some of it information that they prefer not to know? And how could knowledge of a child's possible future risks affect parents' decisions now?
These are just a few of the issues being explored in a grant from the National Human Genome Research Institute, the federal agency that sponsored the Human Genome Project. The Children's Hospital of Philadelphia is one of five U.S. centers, and the only one focusing on pediatrics, to receive a new four-year Clinical Sequencing Exploratory Research Project award. Children's Hospital will receive $2.2 million per year for four years.
Clinical geneticist Ian D. Krantz, MD, is the principal investigator of the project along with Nancy B. Spinner, PhD, director of the Clinical Cytogenomics Laboratory at Children's Hospital.
“By the end of this decade, we anticipate that genomic sequencing will be ready to be offered for the diagnosis of pediatric disorders,” says Dr. Krantz. "Our goal in this research is to help make that information-sharing process systematic, thoughtful and sensitive to the needs and desires of patients and families," adds Dr. Krantz.
Grand Challenges Explorations to Fund HIV Research
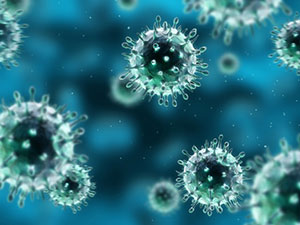
Children’s Hospital recently announced that it will receive funding through Grand Challenges Explorations, an initiative created by the Bill & Melinda Gates Foundation, to fund a new HIV study. Terri Finkel, MD, PhD, chief of Rheumatology and Professor of Pediatrics at the Perelman School of Medicine at the University of Pennsylvania, will pursue an innovative global health research project, titled “Use of a BET Antagonist to Control and Cure HIV Infection.”
Dr. Finkel and her research team will study the potential of drugs called synthetic BET (bromodomain extra terminal) family protein antagonists to control HIV infection and latency. Current HIV drugs reduce the disease-causing virus to undetectable levels, but the virus goes into a dormant state called latency, from which it can make a resurgence if a patient stops taking medication. Previous studies by Dr. Finkel and other scientists have suggested that BET antagonists may flush HIV out of its hiding places where it can be destroyed by other antiretroviral drugs.
Dr. Finkel’s chief collaborator in this project is Gerd A. Blobel, MD, PhD, holder of the Frank E. Weise III Endowed Chair in Pediatric Hematology at Children’s Hospital, who has focused on the biological activities of BET family proteins. In the first part of the project, Drs. Finkel and Blobel will investigate the effectiveness of BET antagonists against HIV in cell cultures. If these results are encouraging, the project will progress to clinical trials in patients with HIV
Grand Challenges Explorations is a $100 million initiative funded by the Bill & Melinda Gates Foundation. Launched in 2008, Grand Challenge Explorations grants have already been awarded to nearly 500 researchers from more than 40 countries.